Abstract
Objectives: To evaluate the antibacterial activity of plumbagin (PGN) against multidrug resistance (MDR) clinical isolates.
Methods: This study was carried out at the Department of Clinical Lab Sciences, King Khalid University from October 6, 2021 to December 14, 2021. We investigated the antibacterial and anti-virulence activity of PGN against MDR Gram-negative (Escherichia coli, Klebsiella pneumoniae, Salmonella Typhi, and Pseudomonas aeruginosa) and Gram-positive (Staphylococcus aureus [S. aureus], Staphylococcus saprophyticus [S. saprophyticus], Streptococcus pyogenes, and Enterococcus faecalis) clinical bacterial isolates. Agar well diffusion, microdilution assay, colony count method, biofilm formation, and time-kill kinetics were employed to probe the MIC, MBC, and anti-virulence activity of PGN.
Results: Plumbagin inhibited the growth of all tested isolates, with S. saprophyticus exhibiting the highest sensitivity. MIC values ranged from 0.029 to 0.117 µg/mL whereas MBC ranged from 0.235 to 0.94 µg/mL, with 79% to 99% growth inhibition. Moreover, all tested isolates showed a marked decrease in biofilm formation, with S. saprophyticus and S. aureus being the most sensitive.
Conclusion: Plumbagin is a stand-alone, broad spectrum antibacterial with promising potential against the rising threat of antimicrobial resistance.
Bacterial infections, such as bacteremia and pneumonia, have been associated with high rates of morbidity, mortality and economic costs, posing a real threat to public health globally. The mainstay of drug therapy for these infections is appropriate antibiotic treatment.1 Over the past 2 decades, however, the level of antimicrobial resistance of major bacterial species, such as Escherichia coli (E. coli) and Pseudomonas aeruginosa [P. aeruginosa], has increased substantially.2 Moreover, multidrug resistance (MDR), defined as resistance to at least one agent in ≥3 antimicrobial groups, has increasingly been reported locally and globally. For instance, a recent study from Saudi Arabia has found that 67% of E. coli urine isolates were MDR.3 Multidrug resistance constitutes a stumbling block in the face of developing new therapeutics. Nevertheless, a combinatorial approach involving the use of established antimicrobial agents along with naturally-derived compounds has produced encouraging results.4
Quinones are aromatic dicarbonyl compounds that naturally exist in plants but can also be synthetically synthesized. Quinones have gained considerable attention for their medicinal properties including antimicrobial, anti-inflammatory, anti-atherosclerotic, and antitumor functions.5 Plumbagin, or 5-hydroxy-2-methyl-naphthalene-1,4-dione, is a yellow crystalline naphthoquinone derivative with a molar mass of 188.17 g/mol. A vitamin K3 analogue, PGN possesses an additional hydroxy group on carbon 5 in the naphthalene group (Figure 1). Plumbagin is present in the root, leaf, and stem bark of multiple plants including Plumbaginaceae, Ebenceae, Dioncophyllaceae, Ancestrocladaceae, Droseraceae, and Juglandaceae families.6,7 In particular, the roots of Plumbago zeylanica L. have been successfully used in India for therapeutic purposes against dermal and musculoskeletal conditions for more than 2500 years.8
- Molecular structure of plumbagin.39 Characteristic hydroxyl and methyl groups added to para-naphthoquinone are noted.
Beside its anti-inflammatory, antioxidant, antitumor, and antidiabetic activities,previous reports have demonstrated the efficacy of PGN against pathogenic microbes.9-12 In particular, Staphylococcus aureus [S. aureus] and Candida albicans were susceptible to PGN in vitro and in vivo.13,14 Plumbagin also exhibited synergistic activity with ciprofloxacin and piperacillin against MDR strains of methicillin-resistant S. aureus (MRSA), which was through inhibition of DNA gyrase.15,9 Nevertheless, the potential efficacy of PGN against other bacterial species remains poorly studied.
Most studies were carried out using plant extracts with mixed chemical constituents, and little is known regarding PGN against MDR bacterial isolates in Saudi Arabia. In this report, we examine the antibacterial activity of PGN against clinical isolates of Gram-negative (E. coli, Klebsiella pneumoniae [K. pneumoniae], Salmonella Typhi [S. Typhi], and P. aeruginosa) and Gram-positive (S. aureus, Staphylococcus saprophyticus [S. saprophyticus], Streptococcus pyogenes [S. pyogenes], and Enterococcus faecalis [E. faecalis]) bacteria in an attempt to further elucidate its potential application in antimicrobial therapy. We also sought to determine the anti-virulence potential of PGN through evaluating its anti-biofilm activity against these isolates.
Methods
This is an experimental investigation that was carried out at the Department of Clinical Lab Sciences, College of Applied Medical Sciences, King Khalid University, Abha, Saudi Arabia, from October to December 2021. Ethical approval was obtained from the Research Ethics Committee at King Khalid University (ECM#2021-2801).
Preparation of PGN stock solution
Plumbagin (CAS #481-42-5) from P. zeylanica L was obtained from Solarbio Life Science (Beijing, China) and the stock solution was prepared by suspending 1.88 µg in 1 mL of dimethyl sulfoxide (DMSO). Stock suspension was sonicated (Sonics Vibra cell; Sonics & Material, Newtown, CT, USA) at 40°C for 10 minutes and aliquots were preserved at –80°C. Working solutions were prepared by taking 1 mL of the stock solution and adding it to 9 mL of 2% DMSO. Further dilutions were made as necessary to achieve the required final concentration of PGN. Appendix 1 shows lack of antibacterial activity by DMSO on tested isolates.
Bacterial strains and growth conditions
A panel of clinical MDR Gram-negative and Gram-positive bacterial strains, including P. aeruginosa, E. coli, K. pneumoniae, S. Typhi, S. aureus, S. saprophyticus, S. pyogenes, and E. faecalis, were used in this study (Table 1). These strains were grown in nutrient broth at 37°C for 24 h.
- Characteristics of bacterial strains used in this study.
Antimicrobial susceptibility testing
A lawn culture of the bacterial inoculum (OD610 of 0.01) was grown on Mueller–Hinton agar (HiMedia Labs, Mumbai, India) with 14 disk antibiotics (Liofilchem, Livorno, Italy) in line with the guidelines of Clinical and Laboratory Standards Institute (CLSI) (16). Following incubation for 24 hours (h) at 37°C, the diameter of the clear zone of inhibition was subsequently measured in millimeters. E. coli ATCC 25922 and S. aureus ATCC 25923 were used as control strains.
Antibacterial susceptibility assay by agar well diffusion
Bacterial strains were grown to the logarithmic phase (OD610 of 0.4-0.6) in nutrient broth and then diluted to a theoretical OD610 of 0.01. Agar well diffusion was used to probe the antibacterial efficacy of PGN.17 Briefly, wells of 6 mm diameter were formed in the nutrient agar using the cap of a sterile syringe and a lawn culture was formed on the agar from diluted cultures using a sterile cotton swab. Next, 20 µL of PGN (1.88 µg/mL) and DMSO were added to triplicate wells and the plates were incubated aerobically for 24 h at 37°C. Gentamicin and vancomycin were used as positive controls and distilled water as a negative control. The diameter of the clear zone of inhibition of bacterial growth including the well diameter was measured in millimeters.
Determination of minimum inhibitory concentration (MIC) and minimum bactericidal concentration (MBC)
Minimum inhibitory concentration and MBC of PGN were determined as described by Wei et al18 with modification. Plumbagin concentrations were diluted 2-fold and ranged from 1.88 µg/mL to 0.014 µg/mL. To determine MIC, cultures were first grown to logarithmic phase and then further diluted in Mueller-Hinton broth to a theoretical OD610 of 0.01. Subsequently, 180 µL of all cultures were transferred to 96-well plates, 20 µL from the 2-fold dilution of PGN were loaded in triplicate wells, and 20 µL of DMSO served as control. Following aerobic incubation for 24 h at 37°C, each well was supplemented with 20 µL of alamar blue (Thermo Fisher, Watham, MA, USA) and observed at 1-hour intervals for the development of a pink color. The lowest concentration that did not cause a color change was recorded as the MIC. For the determination of MBC, 10 µL from wells with no color change were sub-cultured on nutrient agar and incubated aerobically for 24 h at 37°C. The minimum concentration of PGN at which no growth was observed was taken as the MBC for the examined strains.
Growth inhibition
A colony count method was employed to determine the bactericidal effect of PGN. Approximately 10 µL of PGN were added to bacterial suspensions plated on nutrient agar using a spread plate method together with a negative control. The plates were observed for growth inhibition by counting colonies after incubation for 24 h at 37°C. The percentage of loss in viable cells was determined using the following equation:
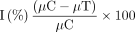
Where I% = percentage of bacterial growth inhibition; µC = mean value of OD610 in control cells; and µT = mean value of OD610 in treated cells.
Antibiofilm effect of PGN
Biofilm formation of the bacterial isolates was evaluated according Zhang et al19 with minor modifications. Briefly, 180 µL of fresh bacterial suspension (OD610=1.0) was added to 20 µL of different concentrations of PGN (MIC x0.5, MIC x1, MIC x2) in a 96-well plate, and the suspension was incubated for 24 h at 30°C without shaking to induce biofilm assembly. Untreated bacterial cells were used in each set of investigations as a negative control. After incubation, crystal violet was added and the absorbance was recorded at 488 nm.
Time-kill kinetics assay
To investigate the effect of PGN on tested bacterial cells over time, 180 µL of the bacterial culture (OD610 of 0.01) was treated with 20 µL of different concentrations of PGN (MIC x0.5, MIC x1, MIC x2). A culture well with 20 µL of DMSO was considered as the control. The plates were further incubated aerobically at 37°C and OD was measured at 610 nm at 2-h intervals. The mean OD readings were plotted against time.
Statistical analysis
Experiments were performed three times, and the results were plotted as mean ± SD. GraphPad Prism 6.0 (GraphPad Software, Inc., San Diego, CA, USA) was used for statistical analysis. Variations between 2 groups were examined with the 2-tailed Student’s t test, while one-way analysis of variance with Dunnett’s correction compared more than two groups. A p-value of <0.05 was considered statistically significant.
Results
Antibiotic susceptibility assay of standard antibiotics
To determine antibiotic susceptibility, clinical isolates of Gram-negative and Gram-positive bacteria including P. aeruginosa, E. coli, K. pneumoniae, S. Typhi and S. aureus, S. saprophyticus, S. pyogenes, and E. faecalis were tested against antibiotics shown in Tables 1 & 2. All of the tested bacterial strains were resistant to 3 or more of the standard antibiotics (Appendix 2 & 3) which indicates that they are MDR. Antibacterial activity of PGN
- Details of antibiotics used in this study.
To assess the antibacterial activity of PGN, clinical isolates of Gram-negative and Gram-positive bacteria including P. aeruginosa, E. coli, K. pneumoniae, S. Typhi and S. aureus, S. saprophyticus, S. pyogenes, and E. faecalis were used. Susceptibility studies showed that PGN had a comparatively higher antibacterial activity against Gram-positive isolates. A zone size above 8 mm was considered significant based on the sensitivity of the bacterial strains to PGN. The zone of inhibition against Gram-positive bacteria was 28 to 35 mm in diameter, while that against Gram-negative bacteria ranged from 17 to 26 mm in diameter (Figure 2A-C).
- Susceptibility of tested organisms to plumbagin (PGN). (A-C) Zone of inhibition is observed on plates supplemented with PGN against A) Pseudomonas aeruginosa, B) Escherichia coli, C) Klebsiella pneumoniae, D) Salmonella, E) Staphylococcus aureus, F) Staphylococcus saprophyticus, G) Streptococcus pyogenes, and H) Enterococcus faecalis. (D & E) MIC and MBC values of PGN against Gram-negative and Gram-positive isolates.
In order to investigate MIC and MBC indices, selected bacterial strains were exposed to the aforementioned volume of PGN followed by an incubation period of 24 h. As shown in Figure 2, all bacterial strains tested were significantly susceptible to PGN. Bacterial growth was inhibited with higher zones of inhibition ranging from 17 to 35 mm, reflective of MIC values of 0.029-0.117 µg/mL and MBC values of 0.235-0.94 µg/mL (Figure 2D and 2E). For comparison, MIC and MBC values of gentamicin and vancomycin were used as controls against Gram-negative and Gram-positive isolates, respectively (Appendix 2).
Plumbagin inhibits the viability of bacterial cells
Growth inhibitory effects of PGN on the tested bacterial strains were determined by counting the bacterial colonies after treating with PGN. The results of colony count on the tested bacterial strains showed a reduction in the number of colonies at the MBC concentration of PGN ranging from 79% to 99% (Figure 3). The lowest growth inhibition was found against S. Typhi (Figure 3A), whereas the highest was recorded against S. saprophyticus (Figure 3B).
- Bacterial growth inhibition by plumbagin (PGN). Mean percentage of growth inhibition + SD by PGN at minimum bactericidal concentration for 24 h at 37°C for (A) Gram-negative and (B) Gram-positive isolates. Control bars indicate all untreated bacterial strains presented as 0% inhibition. CFU: colony-forming unit, P. aeruginosa: Pseudomonas aeruginosa, E. coli: Escherichia coli, K. pneumoniae: Klebsiella pneumoniae, S. aureus: Staphylococcus aureus, S. saprophyticus: Staphylococcus saprophyticus, S. pyogenes: Streptococcus pyogenes, E. faecalis: Enterococcus faecalis
Plumbagin inhibits bacterial biofilm formation
We attempted to investigate whether PGN could inhibit biofilm formation of the tested Gram-negative and Gram-positive bacterial strains. Bacteria were incubated with MIC x0.5, MIC x1, and MIC x2 of PGN for 24 h at 37°C. Furthermore, the rate of biofilm formation inhibition by PGN was also based on the concentration and treatment time.
The inhibition of biofilm formation was significant against all the tested bacteria but it was drastically reduced against S. saprophyticus and S. aureus in the wells treated with MIC x2 of PGN. At MIC x2 of PGN, quantitative estimation of biofilms formed by S. saprophyticus showed a 4.2-fold decrease and by S. aureus a 3.01-fold decrease, compared to control values (Figure 4 A&B). These results suggest that biofilm formation by all the tested bacteria was inhibited by PGN.
- Plumbagin (PGN) reduces biofilm formation. (A) Gram-negative and (B) Gram-positive isolates were treated with different concentrations of PGN (minimum inhibitory concentration [MIC] x0.5, MIC x1 and MIC x2) under biofilm growing conditions for 24 hours and mean absorbance readings at 488 nm + SD were plotted. P. aeruginosa: Pseudomonas aeruginosa, E. coli: Escherichia coli, K. pneumoniae: Klebsiella pneumoniae, S. aureus: Staphylococcus aureus, S. saprophyticus: Staphylococcuss saprophyticus, S. pyogenes: Streptococcus pyogenes, E. faecalis: Enterococcus faecalis
Effect on bacterial growth
To determine time-killing kinetics of PGN, 180 µL of bacterial culture (OD610 of 0.01) were treated with 20 µL of PGN at different concentrations of MIC x0.5, MIC x1, and MIC x2. The growth of bacteria was observed at time intervals of 2 h (Figure 5). It is absolutely apparent that bacterial strain growth was repressed by treatment with PGN at different concentrations. Time killing kinetics indicated a dose dependent bactericidal effect of PGN on the tested bacteria. Our data distinctly indicates a strong bactericidal activity of PGN against the tested bacterial strains.
- Kinetics of plumbagin (PGN)-induced growth inhibition. Representative (A) Gram-negative (a. Pseudomonas aeruginosa, b. Escherichia coli, c. Klebsiella pneumoniae, d. Salmonella) and (B) Gram-positive (a. Staphylococcus aureus, b. Staphylococcus saprophyticus, c. Streptococcus pyogenes, d. Enterococcus faecalis) strains were treated with different concentrations (MIC x0.5, MIC x1 and MIC x2) of PNG. Growth cycle of untreated organisms served as control. OD610 nm was measured at regular intervals of 2 hours and presented as mean + SD.
Discussion
Despite making great strides in the combat against microbial resistance, MDR remains a pivotal challenge in the development and validation of novel antimicrobial therapeutics. This is mainly due to the widespread inappropriate use of current antibiotics, in addition to the stagnated discovery of new efficacious chemotherapeutic agents.9 Although the antimicrobial activity of P. zeylanica extracts is well established in the literature, the antibacterial activity of PGN, a major constituent of this plant, has not been thoroughly investigated. Herein, we demonstrate that PGN exhibits a potent, broad-spectrum antibacterial activity against clinical isolates of major worldwide concern.
The zones of inhibition against Gram-positive bacteria were larger than those against Gram-negative isolates (Figure 2), which could be attributed to the presence of a physical cell wall barrier in the latter as well as the presence of to efflux pumps. This is in parallel to the results obtained by Dissanayake et al9 using P. indica extract. It is worth mentioning that standard antibiotics exhibit MIC values ranging from 15-107 µg/mL20 which, compared to our findings of 0.029-0.117 µg/mL (Figure 2), highlights the potent antibacterial activity of PGN. We have previously shown that thymoquinone had an MIC of 1.04-8.3 µg/mL, reflective of a much weaker activity.4 Furthermore, our data indicate that PGN compares favorably and exceeds in antimicrobial potency a panel of alkaloid, terpenoid, phenolic, and thiophene natural products.21
The highest susceptibility among the tested strains was observed against S. saprophyticus (Figure 2D). This coagulase-negative pathogen is responsible for urinary tract infections (UTIs) and related genitourinary complications in susceptible hosts with preexisting conditions.22 Behind only E. coli, S. saprophyticus is the second most frequently encountered cause of uncomplicated community-acquired UTIs in females.23 Currently, nitrofurantoin and a combination of trimethoprim-sulfamethoxazole are the antimicrobials of choice for S. saprophyticus infections. However, major side effects of these drugs include fatal pulmonary, dermal, gastrointestinal, hematological, and neurological symptoms.24 Thus, in light of available evidence, PGN may offer a safer alternative for S. saprophyticus infections.
Conversely, although shown to be susceptible, E. coli, S. aureus, and E. faecalis required the highest concentrations of PGN compared to the other tested strains (Figure 2 D&E). This is in agreement with the consensus on these organisms being notorious for rapid acquisition of resistance.4 Pathogenic E. coli, including intestinal and extraintenstinal strains, are behind the etiology of diarrhea, UTIs, septicemia, and meningitis, and adhesins, release of toxins, and tissue invasion form the major virulence factors mediating pathogenesis. MDR E. coli strains are responsible for both nosocomial and community-acquired infections, and they are often resistant to front-line agents, such as penicillin and third-generation cephalosporins. This resistance, mediated by extended-spectrum β-lactamase (ESBL),25 has now increased due to the worldwide dissemination of the specific E. coli clone, E. coli ST131.26 In an attempt to tackle this resistance issue, more powerful agents, such as carbapenems and polymyxins, have recently been relaunched as alternatives to inactive antibiotics. However, carbapenems suffer from poor absorbability, and cause pruritis, hepatotoxicity, diarrhea, vomiting, and nausea.27
Virulent strains of S. aureuss cause a variety of fatal pathologies including osteoarthritis, endocarditis, sepsis, and prosthesis-related infections, among others.28 Compared to glycopeptides such as vancomycin, methicillin-sensitive S. aureus responds well to β-lactam antibiotics, but this is not without relapse.29,30 For MRSA, vancomycin and daptomycin remain the gold standard therapy. Nevertheless, the continuing emergence of resistant strains, combined with the established toxicity of these drugs and their prolonged use,underpins the search for alternative, fast-acting, and less harmful alternatives. Importantly, septic arthritis is often managed with immunosuppressants such as dexamethasone, which increases the patient’s susceptibility to infections and predisposes to hyperglycemia, an effect counteracted by PGN.10,31,32
Clinical isolates of E. faecalis cause a host of ailments ranging from UTIs and bacteremia to endocarditis and meningitis. A recognized threat to public health, E. faecalis is most commonly resistant to rifampicin and erythromycin, in addition to vancomycin, which surprisingly may serve as a growth factor.33,34 Enterococcus faecalis is also able to reside in biofilms,35 further aggravating its virulence. Our results indicate that PGN possesses antibiofilm activity (Figure 4). Biofilm formation aids in immune evasion, which fosters prolonged colonization and tissue injury. Moreover, cells embedded in biofilms often remain in a dormant state, having their metabolism operating at a lower rate. As a consequence, biofilm-associated infections often respond variably to available therapy. These infections affect virtually all body systems, and, alarmingly, may promote tumorigenesis.36 Therefore, modulating biofilm assembly and persistence, possibly through adhesins and quorum sensing, may prove to be efficacious against a multitude of life-threatening ailments. Of note, a number of polyphenols, including coumarins, anthocyanins, tannins, and flavonoids have also been demonstrated to interfere with biofilm formation in S. aureus, K. pneumoniae, P. aeruginosa, E. coli, and E. faecalis.37
Development of pharmaceutical formulations relies on interaction of the active ingredient with excipients that could either enhance or suppress its efficacy. Notably, the bark of P. zeylanica has successfully been used to synthesize silver and gold nanoparticles with discernable antibacterial activity against P. aeruginosa and B. subtilis.38 Therefore, evaluating the antimicrobial activity of PGN in nanoparticle formulations, and in the presence of common excipients such as polyethylene glycol, is also warranted.
Study limitations
The current study lacks in vivo confirmation, effects of long-term exposure, and biochemical and molecular mechanisms, in addition to unknown additive or antagonistic interactions of PGN with other antibacterial compounds. Future studies should investigate the possible additive or synergistic role of PGN with standard antibiotics as well as the range of susceptible organisms and the mechanisms involved in its antimicrobial and anti-virulence activities both in vitro and in vivo.
In conclusion, since the majority of previous studies focused on plant extracts with mixed constituents, our report identifies PGN as a stand-alone broad-spectrum antibacterial agent effective against major clinical bacterial isolates from Saudi Arabia. Plumbagin is demonstrated to possess superior potency over numerous established and investigative antimicrobials, in addition to antibiofilm activity.
Acknowledgment
The authors extend their appreciation to the Deanship of Scientific Research, King Saud University, Riyadh, Kingdom of Saudi Arabia for funding this research project through the Vice Deanship of Scientific Research Chairs; Research Chair of Medical and Molecular Genetics. The authors gratefully acknowledge EnglishEdited.com ([email protected]) for English language editing.
Appendix
- Lack of effect of dimethyl sulfoxide on bacterial growth. Zone of inhibition is observed on plates for A) Pseudomonas aeruginosa, B)Escherichia coli, C) Klebsiella pneumoniae, D) Salmonella Typhi, E) Staphylococcus aureus, F) Staphylococcus saprophyticus, G) Streptococcus pyogenes, and H) Enterococcus faecalis.
- Resistance patterns of Gram-negative bacterial strains used in the study.
- Resistance patterns of Gram-positive bacterial strains used in the study.
- Minimum inhibitory concentration (MIC) and minimum bacterial concentration (MBC) values of gentamicin and vancomycin. Gram-negative A) and Gram-positive B) isolates were treated with gentamicin and vancomycin, respectively, to determine MIC and MBC values. P. aeruginosa: Pseudomonas aeruginosa, E. coli: Escherichia coli, K. pneumoniae: Klebsiella pneumoniae, S. Typhi: Salmonella typhi, S. aureus: Staphylococcus aureus, S. saprophyticus: Staphylococcus saprophyticus, S. pyogenes: Streptococcus pyogenes, E. faecalis: Enterococcus faecalis,
Footnotes
Disclosure. This study was funded by the Deanship of Scientific Research through the Vice Deanship of Scientific Research Chairs; Research Chair of Medical and Molecular Genetics, King Saud University, Riyadh, Kingdom of Saudi Arabia.
- Received August 11, 2022.
- Accepted October 20, 2022.
- Copyright: © Saudi Medical Journal
This is an Open Access journal and articles published are distributed under the terms of the Creative Commons Attribution-NonCommercial License (CC BY-NC). Readers may copy, distribute, and display the work for non-commercial purposes with the proper citation of the original work.